So far, we have discussed explosives by when they were invented, and later, to some extent, by their sensitivity to heat, friction, or shock. In this chapter, we will discuss the classes of explosive, which depends on the types molecules used (their chemistry), and the mix of different molecules used. We will be primarily discussing high explosives, since almost all explosives since black powder are of this type.
Nitrates
The nitrate-based explosives contain the nitrate group, NO3. The simplest is ammonium nitrate.

Ammonium nitrate is a salt. A salt is what you get when you combine an acid (in this case nitric acid) with a base (ammonia). In a salt, the ion from the acid is negatively charged, and the ion from the base is positively charged. In a water solution, the water molecules (which have a positive end and a negative end) surround the ions and mask their charges from one another, and they lose their mutual attraction, and dissolve. Take away the water, and the ions arrange themselves in a regular order (thus falling into a lower energy state) and crystallize.
Ammonium nitrate is explosive, unlike metal nitrates such as potassium nitrate (saltpeter). This is because the ammonium ion provides fuel, in the form of those four white hydrogen atoms you can see in the image. When detonated, the reaction products are all gases, such as water vapor, nitrogen gas, and oxides of nitrogen. There is no carbon, so there is no black sooty smoke.
Ammonium nitrate was not used as an explosive before the invention of a way of combining hydrogen with the nitrogen in the air to form ammonia gas. The first process to do that was invented by the German chemists Adolph Frank and Nikodem Caro in 1895. Their process used calcium carbide to react with nitrogen gas at a temperature of 1,000° Celsius. The end product was calcium cyanamide, and the Frank-Caro process is often known as the cyanamide process.
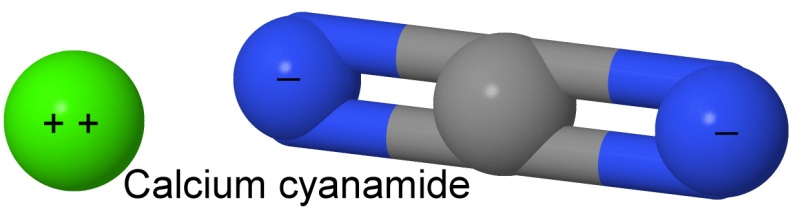
A second process, the Haber-Bosch process, was invented later, and was more economical. Lesser known processes, like the arc process (simulating how lightning makes nitric acid), the nitride process (reacting steam with nitrides), and the Bucher cyanide process (nitrogen, coke, and sodium carbonate react to make sodium cyanide, which steam converts to ammonia) were more costly, and disfavored.
Before these processes were invented, nitrates were mined from dried bird guano deposits in deserts, or leeched from compost heaps.
Nitrogen in the air is very stable. The two nitrogen atoms in N2 are connected by a very strong triple bond, and it takes a lot of energy to tear that bond apart. In nature, this energy comes in the form of lightning. The intense electrical discharge splits the nitrogen molecules, and some of them combine with the second-most plentiful component in air, oxygen. This forms nitric acid, which is quickly diluted by the large amounts of moisture in the air, and comes down as very weakly acidified rain. Plants take up the nitrate ion as fertilizer, and use it to make proteins and other biological molecules.
Nitrates are so important as fertilizer and oxidizer in black powder that the demand began to outstrip the supply in the 1800's. Chemists began looking for ways to "fix" (make available in other forms) the plentiful nitrogen gas in the air.
In the summer of 1909, two German chemists, Fritz Haber and Robert Le Rossignol, demonstrated their way to do this, using high pressure (around 200 atmospheres) and an iron catalyst, promoted by potassium hydroxide. Their laboratory-scale device took an hour to make half a cup of liquid ammonia gas (under pressure to liquefy it). Only about 15% of the original gases combine, so the rest of the gases are cycled through the process repeatedly, until the conversion rate gets to about 98%.
As you would expect from looking at the ammonium ion (or the formula NH4), it takes four times as much hydrogen gas (by volume) as nitrogen gas, to make the resulting ammonia. The reaction is exothermic, meaning it creates heat. Removing the heat allows more of the reaction to go to completion, and fewer of the ammonia molecules to break apart into nitrogen and hydrogen gases. However, cooling the gases makes the reaction go too slowly. The compromise is to keep the temperature at somewhere between 400° Celsius and 450°, which is why only 15% of the reaction completes. Cooling the gases (still under high pressure) then allows the ammonia to be removed, and the remaining gases can once again be heated, producing 15% of ammonia again.
The number of molecules of ammonia is half the number of molecules in the original gases. This means the pressure will drop by half. Dropping pressure favors breaking apart the ammonia to make the original gases again. The answer to this problem is to raise the pressure to 200 atmospheres or so, to move the equilibrium towards forming more ammonia. The higher pressure increases the rate that the molecules react, since they encounter one another more frequently.
High pressures are costly to produce, and require expensive equipment that can handle the pressure. The reaction would go faster at higher pressure, but 200 atmospheres was the sweet spot economically.
As the reaction product is cooled, the pressure would normally drop. Keeping the pressure high allows the ammonia to be liquefied at room temperature, and separated from the unreacted gases, so they can be put through the cycle again.
Haber sold the process to the German chemical company BASF, who put Carl Bosch in charge of scaling the process up to meet industrial demand. He succeeded the following year. Both Haber and Bosch ended up getting Nobel prizes for their work on the Haber-Bosch process.
During World War I, the Allies cut Germany off from the cheap Chilean nitrate sources. The German war effort depended on the Haber-Bosch process to make the explosives needed.
Ammonia can be oxidized to form nitric acid. That acid can be used to make explosives and fertilizer. And, when the acid reacts with yet more ammonia, we get ammonium nitrate, both a fertilizer and an explosive.
Ammonium nitrate is seldom used by itself as an explosive. It has a positive oxygen balance, so adding a fuel makes it a more powerful explosive. Ammonium nitrate mixed with fuel oil (94.3%/5.7%) is a cheap and common high explosive used mostly in mining. The mixture has a low detonation velocity (3,200 meters per second) but produces a lot of gas, giving it what explosives experts call "heave", the ability to move lots of rock. The mixture is known as ANFO, and makes up an estimated 80% of all the explosives use in the U.S. It is not easily detonated, making it what is known as a tertiary explosive, one that is normally set off by a secondary explosive (which is in turn set off by a primary explosive). Adding finely powdered aluminum makes it detonate more easily, and can increase the power by up to 30%. Using nitromethane instead of fuel oil is another way to make the mixture more powerful. That mixture is known as ANNM.
Of course, ammonium nitrate is only the simplest of the nitrate explosives. Cellulose nitrate (guncotton) and glyceryl trinitrate (nitroglycerin) have already been discussed, as have PETN and polyGlyN.
Another simple explosive nitrate is methylammonium nitrate. The extra carbon and hydrogen atoms give it a better oxygen balance than ammonium nitrate, but it is not as cheap. It was used as an explosive by the Germans in World War II, and is now used only in water gel explosives such as Tovex Extra, which have largely replaced dynamite as commercial explosives. The "Extra" is methylammonium nitrate, replacing the more expensive TNT used in the original Tovex. The result is not just cheaper, it has a better oxygen balance, and can be detonated by blasting caps even in narrow drill holes (like the dynamite it replaced).

Water gel explosives are emulsions or slurries of nitrates in water, and they can be used in wet mining situations where other explosives required expensive drying before use. Various ingredients besides the nitrates are added (fuels like nitromethane, powdered coal, aluminum powder, thickening agents, etc.).
Urea nitrate is another simple explosive nitrate. Used as a cheap fertilizer, it is widely available and cheap, making it a favorite of amateur explosive enthusiasts and makers of improvised explosive devices (IEDs) in Pakistan, Iraq, and Afghanistan.
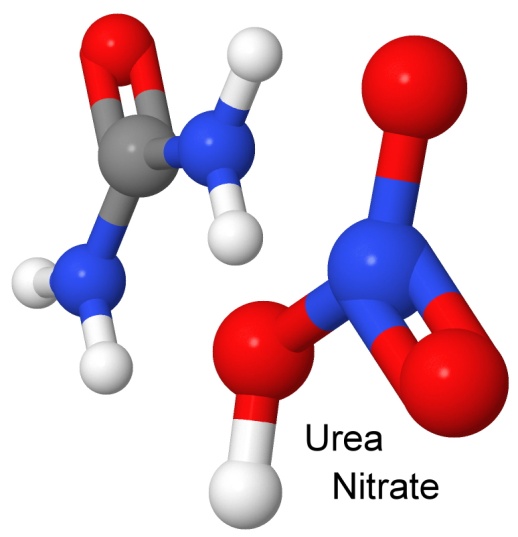
Trimethylolethane trinitrate is similar to nitroglycerin. It is sometimes used in propellants (smokeless powders) instead of nitroglycerin because it erodes gun barrels less, and produces less muzzle flash. However, like nitroglycerin, it is being replaced by less sensitive, safer explosives.
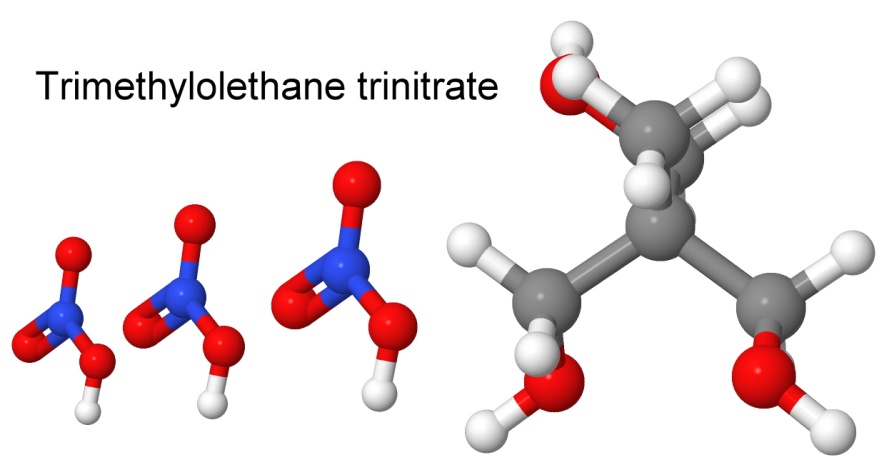
Erythritol tetranitrate is similar to the other tetranitrate we considered earlier, PETN. It has a high detonation velocity (over 8,000 meters per second), but is more sensitive to friction and impact than PETN. It is not used commercially or militarily, but it is a favorite of amateurs, since it is easy to make, and the starting point, erythritol, is a natural sweetener, and easy to find. It has a long shelf life, like PETN. Unlike PETN, it has a positive oxygen balance, meaning extra fuel, such as aluminum powder, or an explosive with a negative oxygen balance, like TNT or PETN, could be added to improve the performance.

Mannitol hexanitrate is a nitrated sugar. It is a powerful secondary explosive (detonation velocity 8,260 meters per second, 1.7 times more powerful than TNT). It is more stable than nitroglycerine, and used as the powder in detonators.

An interesting nitrate is guanidinium nitrate. As a propellant in rockets, it produces a lot of gas at relatively cool temperatures. It is used in toys as the propellant in Jetex engines.

It is also known (incorrectly, but widely) as guanidine nitrate. Completing the circle, it can be made by reacting calcium cyanamide with ammonium nitrate.
Nitrides
Another class of explosives is the nitrides. The "fulminating silver" discussed earlier is silver nitride, Ag3N. It explodes violently, leaving metallic silver and nitrogen gas. Silver nitride is formed when an excess of aqueous ammonia (NH4OH) is added to silver nitrate and allowed to stand (the reaction goes through stages, first producing silver oxide, which reacts with the extra ammonia to form diamine silver hydroxide, which gradually decomposes to form silver nitride.
Another very sensitive explosive nitride is mercury(II) nitride. Made by adding mercury oxide to aqueous ammonia at 10° Celsius and then heating, it also explodes quite violently, from heat of just a slight touch. Nitrides are often too sensitive to be useful explosives.
A third class of explosives is the fulminates. We have discusses mercury fulminate, which found wide use as a primary explosive in guns (it is now illegal in most countries). Platinum fulminate is also explosive, and, as you can imagine, a little too expensive to find wide use. It was discovered by Edmund Davy, the cousin of Sir Humphry Davy, the person who damaged his eyes while experimenting with explosive nitrogen chloride. Edmund Davy also discovered acetylene, and started the practice of bolting zinc onto ship's bronze rudders to prevent corrosion.
Potassium fulminate is another primary explosive that found use in early percussion caps. It is less sensitive than mercury fulminate. Silver fulminate is so sensitive it will go off under its own weight if too much is stored at a time. It is used in firework toys called bang-snaps, where a little of it (80 micrograms) is mixed with sand and wrapped in tissue paper. On hitting the ground, or being rubbed between the fingers, a loud snap occurs.
The acetylene that Edmund Davy discovered acts as a weak acid. It will combine with some metals to make explosive acetylides. We have already discussed copper acetylide and silver acetylide. Silver acetylide is formed by bubbling acetylene gas through a solution of silver nitrate. That process was discovered in 1866 by the famous French chemist Marcellin Berthelot.
Nitro
The nitro group (NO2) forms a large group of famous explosive compounds. We have discussed TNT, TATB, TNP, nitroguanidine, and others. Some other nitro compounds are nitromethane and nitroethane, used in racing fuels, or mixed with ammonium nitrate to form explosives.

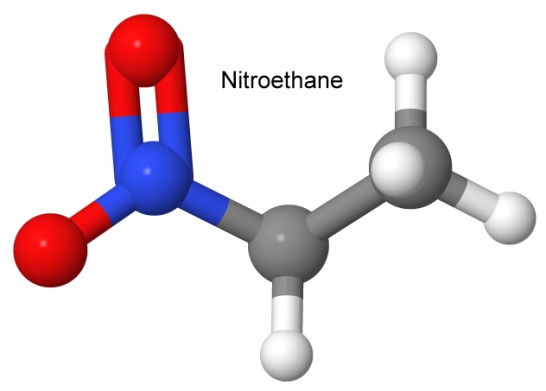
Note the difference between the nitro group and the nitrate group (the latter has an extra oxygen). Many nitrate explosives have common names that make them sound like they are actually nitro explosives (nitrocellulose, nitroglycerin, nitrostarch, etc.). You can look at the structural formula to quickly tell the difference.
Another nitro explosive is nitrourea.

While nitrourea is a respectable explosive, it degrades quickly in contact with moisture, and is not used commercially as an explosive.
More than one nitro group can be added to a molecule. One of the early explosives having two nitro groups is diazodinitrophenol (DDNP, or Dinol). It was discovered in 1858 by Peter Griess, (who later discovered lead styphnate, another primary explosive). His discovery of the diazonium salts (of which DDNP is one) made him famous in chemistry circles, and got him a job in England at the Royal College of Chemistry. Diazonium salts are widely used in making dyes.

DDNP is a less sensitive primary explosive than mercury fulminate, and is thus somewhat safer to use. It is also more powerful, allowing it to set off the new less sensitive secondary explosives more easily. When unconfined, it simply burns, with a quick flash, like guncotton. If confined, as in a firecracker, it can be set off with a burning fuse. Since DDNP contains no lead, it is less toxic than lead azide or lead styphnate, and has been substituted for those in primers and detonating caps.
Adding three nitro groups to a molecule is more difficult, because the nitro groups do not respond well to crowding. However, the class of explosives containing three nitro groups is one of the largest, and includes TNT, RDX, Picric Acid, Dunnite, and Tetryl. Another notable trinitro explosive is lead styphnate, mentioned several times earlier, as a common primary explosive.

One very interesting nitro group explosive is octanitrocubane, or ONC. The molecule cubane is fascinating all by itself. It is a cube of carbon atoms, each one bonding to another carbon with three bonds, and to a hydrogen atom with the remaining bond. It was first synthesized by Philip Eaton of the University of Chicago in 1964, in a remarkable 15-step sequence.
Cubane is sometimes called the "impossible" molecule, since the 90-degree bond angles of the eight carbons are extremely strained. We have seen before how the energy stored in such strained bonds help molecules to rearrange, releasing that energy to add to the explosive force. Bond angles between three carbon atoms are most relaxed at 109.5°, almost 20° more relaxed than the tightly wound cubane is.
Since the original 15-step synthesis, several improved methods with fewer steps have been developed. Cubane is now being produced in multi-kilogram quantities by Fluorochem in California, but is still quite expensive.
Despite cubane's strained bonds, it is highly stable. This is because there are no kinetically viable paths by which cubane can rearrange thermally. Just breaking one bond makes only a small change in the structure, and the result is still quite strained. It takes over 230° Celsius before cubane decomposes, and even then, it does so slowly.
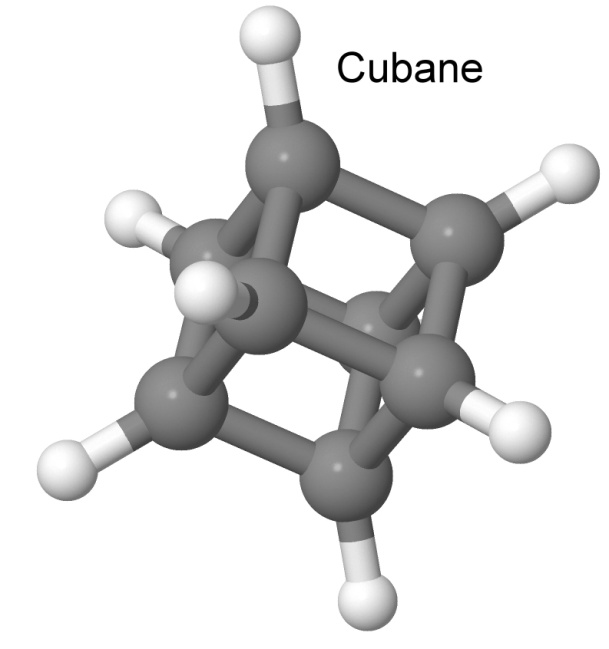
Eaton did not stop there. Because cubane is very dense for a hydrocarbon (1.979 grams per cubic centimeter, so it sinks in water), and it has highly strained bonds, the US Army Armament Research and Development Center came to Eaton with a proposal. They wanted him to create an explosive out of the molecule, by adding eight nitro groups to it, one at each corner of the cube.
The resulting molecule would have some interesting properties. Since there is no hydrogen, it would explode to create eight carbon dioxide molecules and four nitrogen gas molecules, but no water. Thus there would be no smoke or steam produced, making it an excellent propellant for a rocket (no telltale plume behind it, making it difficult to track). The resulting gas would expand to 1,150 times the volume of the original explosive. The energy release would be phenomenal, and it would have a perfect oxygen balance, with no remaining fuel left over after the explosion, and no remaining oxygen.
In addition to being very powerful, octanitrocubane would be an insensitive explosive, so safe to handle you could hit it with a hammer and it would not explode, and light it with a match and it would just burn, without detonating. It was predicted to be less shock sensitive than CL-20. When a nitro group is attached to a carbon (as it is in TNT), the molecule is more stable than when it is attached by way of an oxygen (as it is in nitroglycerin), or when it is attached by way of a nitrogen (as it is in RDX, HMX, and CL-20).

The density of octanitrocubane helps to increase the detonation velocity. So does the high weight of the product gases (CO2 weighs 44 atomic mass units, and N2 weighs 28, while H2O only weighs 18). The high number of reactant gas molecules is a third factor. The high amount of energy released is the fourth part of the equation for calculating the detonation velocity, so octanitrocubane wins on all counts.
The detonation pressure is also important in an explosive, and it goes up with the square of the density. Octanitrocubane wins again. No other combination of carbon, nitrogen, and oxygen has a density as high as octanitrocubane. Compared to TNT, octanitrocubane is 2.38 times more powerful.
There is at least one more powerful explosive, however. The step just before octanitrocubane produces heptanitrocubane. It has one hydrogen atom. It is not as oxygen balanced as octanitrocubane. It is not so beautifully symmetrical. But it has one advantage that tips the balance. It is a little bit denser (2.028 grams per cubic centimeter). And, because the detonation pressure is a factor of the density squared, that makes enough of a difference to make heptanitrocubane the winner. This may not last long, however, as theoretical calculations show that there should be a crystal form of octanitrocubane that has a density of 2.1 grams per cubic centimeter. That crystal structure is different from the one currently being tested, and crystallizing the compound in some other way (different solvents, temperatures, or under pressure) may be the key to getting the higher density. The density of the densest form of CL-20, by comparison, is 2.044, which is denser still (but it is not oxygen balanced, as it has 11% too little oxygen).
Octanitrocubane is currently fabulously expensive to make. One of the cheapest cubane compounds is sold for $40,000 a kilogram, and octanitrocubane is harder to make than that. But theoretical calculations show that there might be a direct synthesis path to octanitrocubane by combining four dinitroacetylene molecules. This synthesis releases energy, so it should be chemically favorable. There is one catch — at the time of this writing, no one has yet made any dinitroacetylene.
Nitramines
A subclass of nitro group explosives is the nitramines. Nitramines (or nitroamines) are a nitro group connected to a nitrogen atom. The simplest member of the group is nitramide, where the extra nitrogen has two hydrogen atoms to complete the molecule.

Several of the most powerful and prominent military explosives are nitramines, such as RDX, HMX, Tetryl, nitroguanidine, and CL-20.

Some of the new nitramine explosives combine the stabilizing effect of pyridine (the central ring in the explosive PYX) with nitramines, to get an insensitive explosive with the power of HMX.
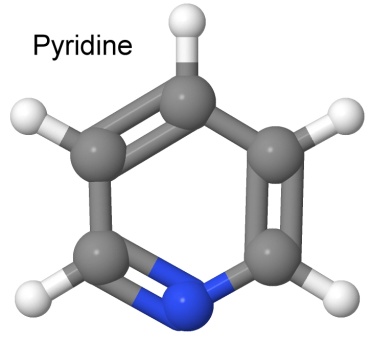
One of those new explosives is diaminodiaminotrinitropyridine, or DADNPO.
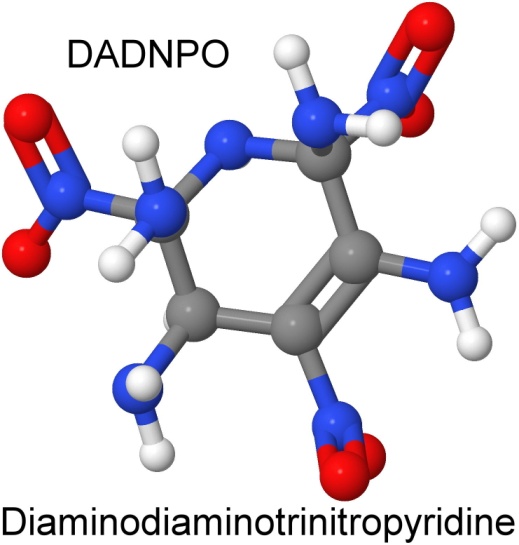
On October 10, 1989, Thomas G. Archibald, working at explosives maker Fluorochem, Richard Gilardi (Naval Research Laboratory), K. Baum (Fluorochem), and Clifford George (NRL) described a new nitramine explosive in the Journal of Organic Chemistry. Perhaps the simplest example of a nitramine, the compound, called trinitroazetidine, or TNAZ, is a high energy density molecule with a high oxygen balance. (Gilardi worked with Philip Eaton on octanitrocubane.)
On June 7, 1993, Michael A. Hiskey and Michael D. Coburn filed a patent for making TNAZ from inexpensive ingredients, with better yields. Not to be outdone, Archibald and three other chemists filed a patent on May 15, 1995 for a process that has high yields, and uses "mild conditions".

TNAZ has nearly the energy density (96%) of HMX, and melts without decomposing at the temperature of boiling water, making it melt-castable, like TNT (but with half-again as much power). Because it has a high oxygen balance, and the reaction gases are very hot, it is expected to do well in composite explosives. While the shock and friction susceptibilities are similar to other nitramines, the TNAZ is significantly less heat sensitive than RDX, HMX, or CL-20. No detonation occurred at temperatures below 199° Celsius.
As a molecule with strained bonds caused by the four-atom ring, TNAZ has extra stored energy that is released when it detonates or burns, like CL-20 and octanitrocubane. Other selling points for TNAZ as an explosive or as a propellant is that it does not attract moisture, and is compatible with aluminum, brass, steel, and glass, unlike more chemically reactive explosives.
While the nitramines are generally very high energy density molecules, the record holder is another nitro group explosive, hexanitrobenzene, or HNB.
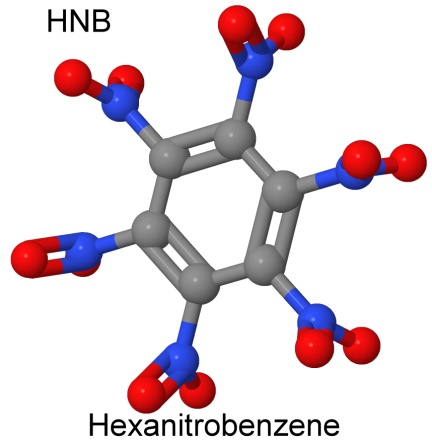
Hexanitrobenzene was first formulated in Germany during World War II, but it is too sensitive to light to be used in commercial or military explosives.
The following table shows some of the higher density explosives and compares then to HMX:
HNB |
1.92 grams/cc |
1650 calories/gram |
3251 calories/cc |
115% of HMX |
CL-20 |
1.96 grams/cc |
1500 calories/gram |
3060 calories/cc |
109% of HMX |
HMX |
1.89 grams/cc |
1480 calories/gram |
2816 calories/cc |
100% of HMX |
TNAZ |
1.83 grams/cc |
1465 calories/gram |
2710 calories/cc |
96% of HMX |
PETN |
1.73 grams/cc |
1490 calories/gram |
2652 calories/cc |
94% of HMX |
TNT |
1.53 grams/cc |
1090 calories/gram |
1803 calories/cc |
64% of HMX |
Sometime in the 1970's, Soviet chemists developed a new explosive, called ammonium dinitramide. This discovery remained secret until the molecule was rediscovered in the US in 1989. On June 18, 1990, Jeffrey C. Bottaro and three other chemists at SRI International filed a patent on it. The molecule, nicknamed ADN, is a powerful oxidizer, and is being considered for new rocket propellants as a replacement for the troublesome ammonium perchlorates currently in use.
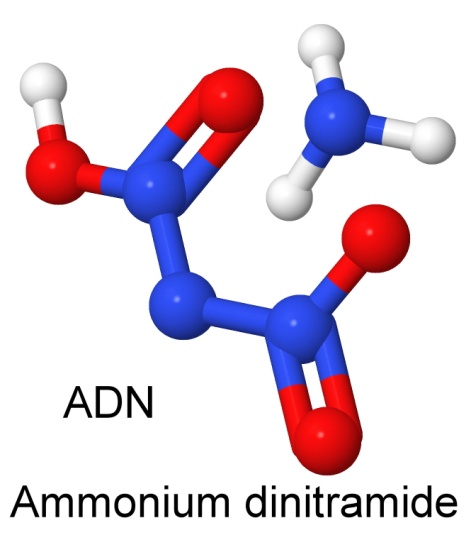
Amines, azides, and nitrides
The amines are another class of explosives with a large number of compounds. We have already discussed some special cases of amines, such as the azides, and the tertiary amines nitrogen trichloride and nitrogen triiodide. Nitrogen tribromide is another tertiary amine. Selenium nitride SeN is a highly explosive red powder. A yellow form, Se2N2 is also explosive, detonating at 200°. Also explosive are Se4N4 and Se4N2. None of them have found any commercial or military uses.
Sulfur also has explosive nitrides, notably disulfur dinitride, S2N2, and tetrasulfur tetranitride, S4N4. Both have interesting structural formulas:
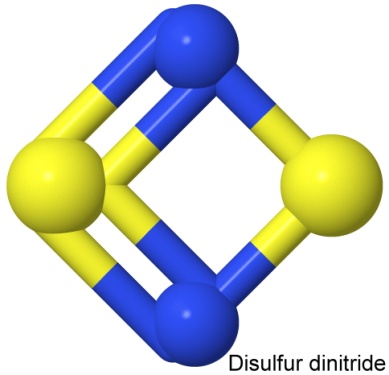

Each of these compounds can have either the sulfur or the nitrogen first in the name, so disulfur dinitride is also dinitrogen disulfide.
We have discussed lead azide, silver azide, and sodium azide earlier. They are all sensitive primary explosives, used in percussion caps and airbags. Cyanuric azide is a heat-insensitive primary explosive with a respectable detonation velocity of 7,300 meters per second, similar to nitrocellulose or picric acid.
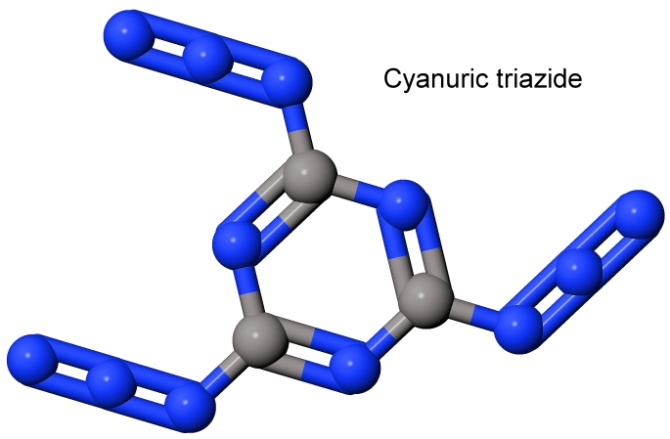
It is extremely shock sensitive, and the reaction products are graphite and nitrogen gas.
Chlorine azide is so unstable it can spontaneously detonate even when very cold. This makes it useless. But as a bookend to nitrogen trichloride, I include it here:

Other uselessly sensitive explosive azides are copper(II) azide, ethyl azide, the gas fluorine azide, silicon tetraazide, and tetraazidomethane.
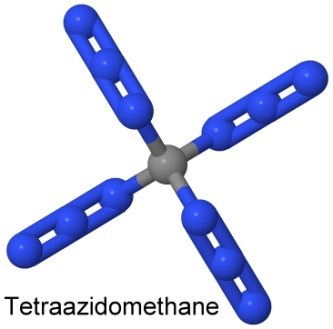
Another sensitive azide is 1-(5-tetrazolyl)-3-guanyl tetrazene hydrate, known as "tetrazene explosive" to distinguish it from the simpler, non-explosive molecule tetrazene. It is more sensitive than mercury fulminate, and like that compound, it is used as a primary explosive in detonators. It is not to be confused with tetracene.


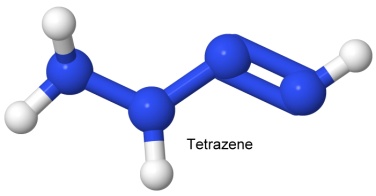
In 1999, chemist Karl Otto Christe created the first new all-nitrogen molecule in over a century. The new molecule, N5+, is the third all-nitrogen species known, after N2 (the nitrogen gas in the air), and azide, N3-, the part of azides that makes them go bang. N5+ is now known as pentazenium.
Christe was working for the Air Force Research Laboratory at Edwards Air Force Base in California. The lab was working on alternatives to hydrazine, the highly toxic propellant used in many of the Air Force rocket engines. Not at all stable by itself, pentazenium combines with antimony hexafluoride to form N5+SbF6-, which is shock-resistant and heat stable up to 60° Celsius.

Finally, now that cubane has been synthesized, and octanitrocubane produced, the challenge for chemists is to come up with the next all-nitrogen species that might actually be stable, octaazacubane. It has not (as of this writing) been synthesized.

Similarly, an all-nitrogen version of buckminsterfullerene, N60 would be a very high-density, possibly insensitive, high explosive that would produce only nitrogen gas as a reaction product. It is on a lot of wish lists.

Peroxides
The next group of explosives is the peroxides. We have discussed triacetone triperoxide (TATP), benzoyl peroxide, peroxymonosulfuric acid, and methyl ethyl ketone peroxide (MEKP) earlier. Like them, the molecule hexamethylene triperoxide diamine (HMTD) is an unstable primary explosive that easily detonates with heat, impact, or friction. Because it is easy to make, it is used by suicide bombers and other terrorists (or would-be terrorists). It may be that once you have decided on suicide, the timing is not that important.
Furazans and furoxans
The molecule furazan (1,2,5-oxadiazol) has some nice properties as a starting point for building a new explosive molecule.
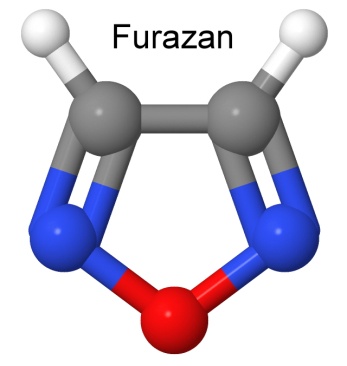
It is flat, which means it can pack more easily in a crystal, giving it a high density. It is stable. Ring molecules with alternating double and single bonds share the electrons between those bonds, so it acts as if each bond has one and a half electrons. This makes these "aromatic" rings more stable than other structures. The oxygen atom in the furazan is not bonded to carbon, but to two nitrogen atoms. This is a weaker bond, allowing the oxygen to free up in an explosion, and combine with hydrogen or carbon, producing energy. The two nitrogen atoms pair up to form nitrogen gas.
Furazan was first synthesized in 1964. By 1968, Michael Coburn (the creator of the explosives NTO, PATO, PYX, and ATNI) had synthesized several furazan compounds, such as DAF, or diaminofurazan. Other chemists added to the furazan list, creating ANF, DAAF, and DAAzF in 1981, and DNFX in 1994.



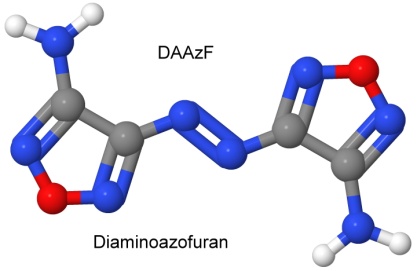


DAAF is used as a ballistic modifier in ammonium perchlorate composite propellants (solid rocket fuels). As DAAF decomposes, it releases ammonia, which slows the burning rate of the ammonium perchlorate fuels. DAAF is also being investigated as a less sensitive replacement for PBXN-7 (60% TATB, 35% RDX, 5% Viton) and PBXW-14 (50% HMX, 45% TATB, 5% Viton). Matching the shock sensitivity of these explosives was an important factor.
DAAF is also being looked at in castable replacements for Composition B (RDX and TNT) for environmental reasons. Its synthesis is environmentally friendly, and it is suitably insensitive, while acting in other ways identically to Composition B when it is combined with melt-castable low melting point explosives like ammonium nitrate and urea (in a combination with a lower melting point than either ingredient alone — called a eutectic mixture).
Furazans can be oxidized to create furoxans. DNFX is a furoxan. It is fully nitrated and highly oxidized. DNFX is a liquid that is stable at -20° Celsius, but decomposes slowly at room temperature. It is very sensitive, and must be handled carefully. However, as a starting point for other furoxan explosives, it has reactive nitro groups that can be substituted, making larger, more stable molecules.
One very energetic furoxan is dinitrodiazenofuroxan, or DNAF. With a detonation velocity of 10,000 meters per second, it may be the current record-holder for an energetic molecule, on a comparative basis with CL-20 and octanitrocubane. But it has a high impact sensitivity, and decomposes at 127° Celsius, so as a practical explosive, it does not compare to those two.
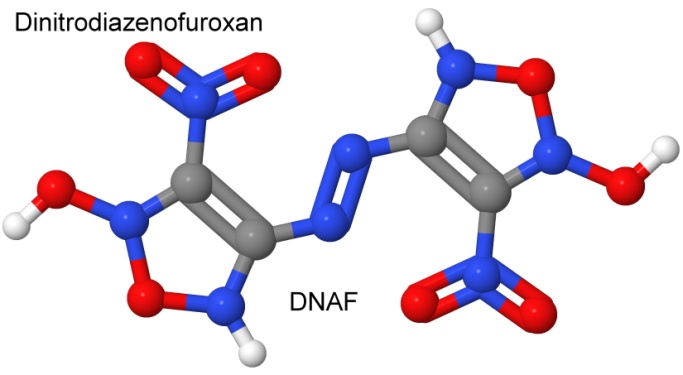
As an example of a typical furazan, let's look at the molecule dinitrohydrazinofurazan.
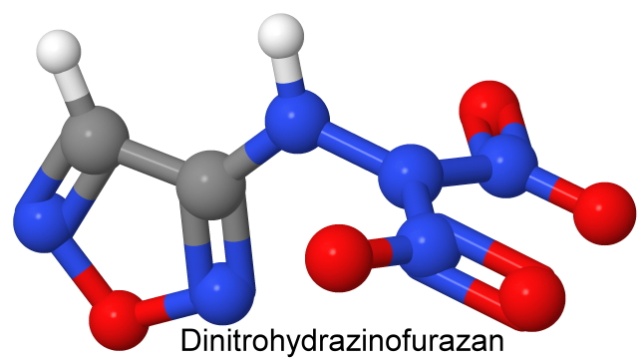
It has two carbon atoms, two hydrogen atoms, five oxygen atoms, and six nitrogen atoms. Using our earlier rule of thumb for figuring out what the reaction products are, we will first remove all the nitrogen atoms and pair them up. There are three pairs. Next, we take pairs of hydrogen atoms and add an oxygen atom to each one, to form water vapor. There are only two hydrogen atoms, so we only have one water molecule. Now we take the carbon atoms and add an oxygen atom to each one, to make carbon monoxide. We have two oxygen atoms left over, so we can use them to oxidize the carbon monoxide molecules to carbon dioxide.
One molecule of dinitrohydrazinofurazan will release three N2 molecules, one water molecule, and two carbon dioxide molecules. There is nothing left over. It has a perfect oxygen balance.